Vertically stacking two-dimensional (2D) materials to form van der Waals homo- or hetero-structures has become an effective means for regulating their physical and mechanical properties. In particular, when a small twist angle is present at the stacked interface, the 2D structures often show many interesting and even magical physical phenomena owing to the unique interlayer coupling.
In the case of bilayer graphene with a small twist angle, the twisted interface will undergo spontaneous atomic reconstruction due to the competition between the interlayer stacking energy and the intralayer elastic strain energy. This special stacked structure can lead to many unexpected phenomena, including Mott insulating state, unconventional superconductivity and spontaneous ferromagnetism.
Recently, it has been found that twisted interfaces can not only appear in the surface layer, but can also be embedded inside the van der Waals structures, which may lead to richer physical behaviors. For these interesting 2D architectures, their physical properties are highly sensitive to the stacking state of the internal layers and interfaces.
Unfortunately, how to precisely characterize the embedded stacking structure is still a great challenge. In addition, whether the embedded twisted interfaces would also undergo atomic reconstruction and what impacts the reconstruction may have on the neighboring atomic layers as well as the whole stacked units are scientifically intriguing and remain unexplored.
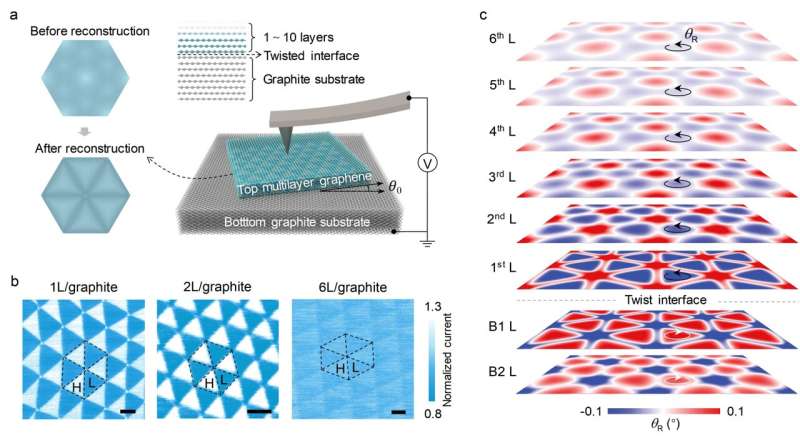
To answer these questions, Professor Qunyang Li’s group at Tsinghua University and Professor Ouyang Wengen’s group at Wuhan University have developed a new method based on conductive atomic force microscopy (c-AFM) to characterize and reconstruct the internal stacking state of twisted layered material through simple surface conductivity measurements. The related work has been published in National Science Review.
Their experimental results have shown that the twisted interfaces can still undergo atomic reconstruction and notably affect the surface conductivity even when they are embedded 10 atomic layers beneath the surface. To better understand the atomic structure of the twisted multilayer system, a multilayer graphene system similar to the experimental samples has been constructed in a molecular dynamics (MD) simulation model by accurately considering the interlayer interactions.
The simulation results have revealed that for small-angle twisted interfaces embedded in the interior of material, atomic reconstruction can indeed occur and promote the in-plane rotational deformation of the adjacent graphene layers. However, the atomic rotational deformation of graphene layer gradually decays as one moves away from the twisted interface.
Based on the atomic structures revealed in MD simulations, the research group proposed a series spreading resistance model (SSR model) to quantify the influence of the stacking state of twisted multilayer system on its surface conductivity.
The new model enables a correlation between the surface conductivity and the internal stacking structure to be made directly, which is applicable even for twisted multilayer samples with complex crystal defects (e.g., dislocations). The work provides a simple, convenient and high-resolution means to characterize the internal stacking structures of twisted layered materials, which is crucial for fundamental studies of 2D stacked structures and the development of emerging twisted electronics.