Materials scientists have extensively studied fast ion permeation in nanofluidic channels in the past decades due to their potential within filtration technologies and osmotic energy harvesting. While the mechanisms underlying ion transport have yet to be understood, the process can be achieved in nanochannels developed in a carefully regulated manner.
In a new report now published in Science Advances, Yu Jiang and a research team in physical chemistry of solid surfaces in China described the development of two-dimensional nanochannels with their top and bottom walls containing atomically flat graphite and mica crystals.
The distinct wall structures and properties allowed the investigation of interactions between ions and interior surfaces. The team noted enhanced ion transport within the channels that are orders of magnitude faster than in bulk solutions, providing insights into surface effects on ion transport at the nanoscale.
Nanoscale ion transport
Mechanisms of nanoscale ion transport can outperform their macroscale counterparts due to their transport rates. Examples include fast ion flow through protein channels in cell membranes in a process that is critical for the essential functioning of life. These include ion permeation through nanoporous membranes for water purification, ion separation and osmotic power generation. To understand the mechanisms of fast ion transport at the nanoscale, researchers must create nanochannels with well-regulated geometry and interior structures.
Yu Jiang and team investigated the origin of fast ionic transport within nanochannels containing ion adsorption sites in the interiors. The simplified design minimized the chance of contaminating channel interiors with chemicals and polymers during fabrication to study adsorption effects on pristine surfaces.
During the experiments, Jiang and colleagues assembled mechanically exfoliated graphite and mica crystals and transferred them to an aperture on silicon substrates. They aligned the graphite/mica heterostructures with the aperture for the top graphite layer cover, while the bottom layer aligned with the aperture at their edges as determined by the transfer method.
The scientists used an atomic force microscope to measure the thickness of the top graphite on mica in aqueous solutions. They then measured the mean height of mica and graphite surfaces in the channel region. Since graphite and mica layers can delaminate at high salt concentrations of 2 M with relatively large ionic currents through the channels, they used solutions with salt concentrations equal to or smaller than 0.1 M for experimental accuracy.
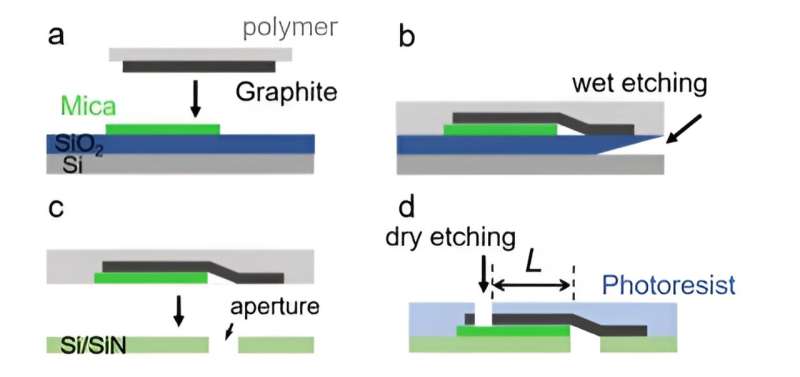
Additional experiments
The scientists estimated the effective height of the channels seen by ions and confirmed the height characterized by atomic force microscopy. During the experiments, they filled the two reservoirs with various chloride solutions of 0.1 M and 0.01 M concentrations, respectively, to create a concentration gradient.
Jiang and colleagues studied the surface effects of the channel’s interior upon ion transport and measured the ionic conductivity of potassium chloride as a function of its bulk concentration. The team investigated the ion transport process in the G-mica channels and narrowed the number of possible mechanisms by performing additional measurements.
Outlook
The high conductance and selective ion adsorption on mica surfaces indicated considerable surface diffusion. The scientists introduced a quantitative expression for ion transport in the graphite-mica channels to provide insights to related mechanisms.
They described the surface conductivity to be due to the migration of adsorbed cations while considering the effective surface salt number density, the surface mobility of adsorbed cations, and focused on the transport of monovalent cations. The relatively large adsorption energy of cations limited their desorption, before migration to highlight the importance of mica for ion transport.
In this way, Yu Jiang and colleagues highlighted surface diffusion as an additional ion transport path in nanofluidics to provide ionic conductivity that are orders of magnitude higher than in bulk solutions. The value is among the highest reported from single nanochannels. The capacity to create channels using mica group crystals that have preferences of adsorbing diverse cations can distinguish ions that depend on their adsorption energies for ion transport and sensing applications.