Researchers at the Paul Scherrer Institute PSI have been improving the resolution of a process known as photolithography. They hope to use their technique to help advance the miniaturization of computer chips.
Miniaturizing computer chips is one of the keys to the digital revolution. It allows computers to become ever smaller and, at the same time, more powerful. This in turn is a prerequisite for developments such as autonomous driving, artificial intelligence and the 5G standard for mobile communications. Now a research team led by Iason Giannopoulos, Yasin Ekinci and Dimitrios Kazazis from the Laboratory of X-ray Nanoscience and Technologies at the Paul Scherrer Institute PSI has devised a technique for creating even denser circuit patterns.
The current state-of-the-art microchips have conductive tracks separated by twelve nanometers, i.e. about 6000 times thinner than a human hair. The researchers, by contrast, have managed to produce tracks with a separation of just five nanometers. As a result, circuits can be designed much more compactly than before.
“Our work showcases the patterning potential of light. This is a significant step forward for both industry and research,” explains Giannopoulos.
Microchips are produced like the pictures on a cinema screen
As recently as 1970, there was only room for around 1000 transistors on a microchip. Today, an area barely larger than the tip of a finger can hold about 60 billion components. These components are manufactured using a process called photolithography: a thin slice of silicon, the wafer, is coated with a light-sensitive layer, the photoresist.
It is then exposed to a pattern of light corresponding to the blueprint for the microchip, which alters the chemical properties of the photoresist, making it either soluble or insoluble to certain chemical solutions. Subsequent treatment removes the exposed (positive process) or unexposed (negative process) regions. In the end, conductive tracks are left behind on the wafer forming the desired wiring pattern.
The type of light used is crucial for miniaturization and for making microchips more and more compact. The laws of physics dictate that the smaller the wavelength of the light used, the more closely the structures in the image can be packed. For a long time, the industry used deep ultraviolet light (DUV). This laser light has a wavelength of 193 nanometers. By comparison, the range of blue light visible to the human eye ends around 400 nanometers.
Since 2019, manufacturers have been using “extreme ultraviolet light” (EUV) with a wavelength of 13.5 nanometers in mass production, more than ten times shorter than before. This makes it possible to print even finer structures, down to ten nanometers and less. At PSI, researchers use radiation from the Swiss Light Source SLS for their investigations, tuned to 13.5 nanometers in accordance with the industry standard.
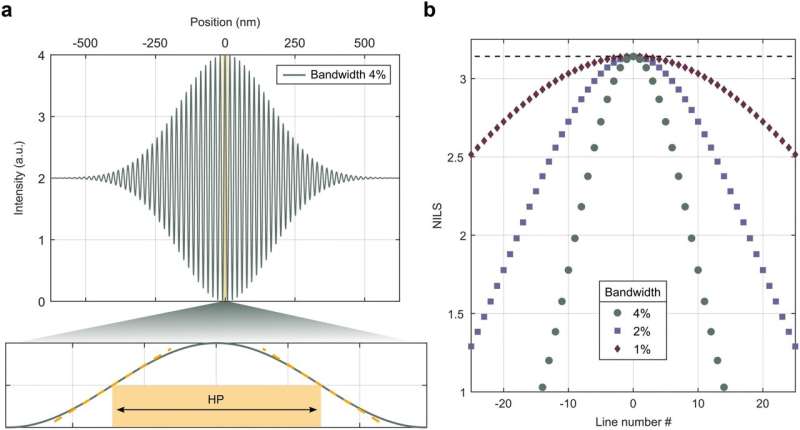
Photon-based lithography can achieve very high resolution
However, the PSI researchers extended conventional EUV lithography by exposing the sample indirectly rather than directly. In EUV mirror interference lithography (MIL), two mutually coherent beams are reflected onto the wafer by two identical mirrors. The beams then create an interference pattern whose period depends on both the angle of incidence and the wavelength of the light.
The group was able to achieve resolutions, i.e. track separations, of five nanometers—in a single exposure. Viewed under an electron microscope, the conductive tracks were found to have high contrast and sharp edges.
Kazazis notes, “Our results show that EUV lithography can produce extremely high resolutions, indicating that there are no fundamental limitations yet. This is really exciting since it extends the horizon of what we deem as possible and can also open up new avenues for research in the field of EUV lithography and photoresist materials.”
A new EUVL tool from the end of 2025
At the moment, this approach is not interesting for industrial chip production, because it is very slow compared to industrial standards and can produce only simple and periodic structures rather than a chip design. However, it offers a method for the early development of photoresists needed for future chip production with a resolution that is not possible in the industry.
The team plans to continue their research using a new EUV tool at the SLS, expected by the end of 2025. The new tool coupled with the SLS 2.0, which is currently going through an upgrade, will provide much enhanced performance and capabilities.