Active matter systems feature unique behaviors that include collective self-assembly structures and collective migration. However, the efforts to realize collective entities in spaces without wall-adhered support, in order to conduct three-dimensional locomotion without dispersion, are challenging.
In a new study, published in Science Advances, Mengmeng Sun and a research team in mechanical engineering and physical intelligence in China and Germany, were bioinspired by migration mechanisms of plankton and proposed a bimodal actuation strategy by combining magnetic and optical fields.
While the magnetic field triggered the self-assembly of magnetic colloidal particles to maintain numerous colloids as a dynamically stable entity, the optical fields allowed the colloidal collectives to generate convective flow through photothermal effects for 3D drifting. The collectives performed 3D locomotion underwater to provide insights into the design of smart devices and intelligent materials for synthetic active matter that can regulate collective movement in 3D space.
Active living matter
Active living matter is ubiquitous in nature, offering self-assembled collectives that can accomplish complex tasks that surpass individual capabilities, which include bird flocks, and colonies of bacteria.
Bioinspired by natural collectives, it is possible to examine colloids as building blocks for materials, much like atoms that form building blocks of molecules and crystals. Colloidal self-assembly can be studied as a method to fabricate nanostructures with technical implications to build nanoscale electronics, energy conversion or storage, drug delivery and catalysts.
The process of colloidal assembly can be guided on a patterned substrate or through Langmuir-Blodgett assembly, for assembly in fibers and cells, and as chemical signals.
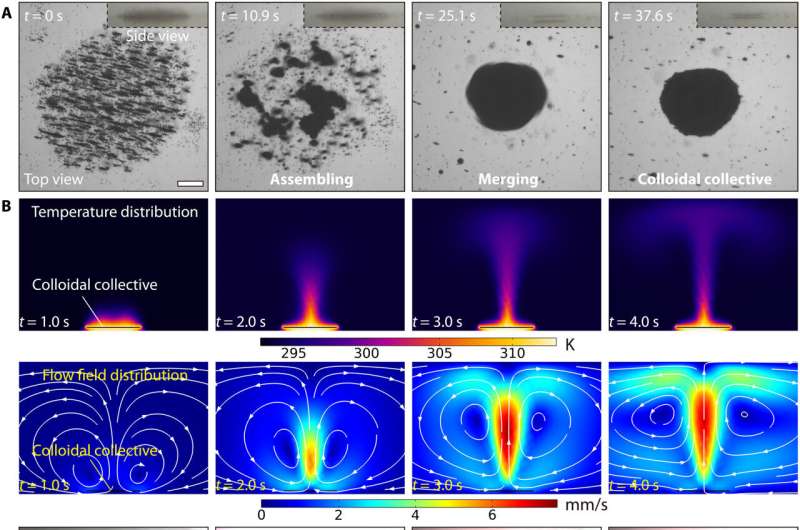
In this work, Mengmeng Sun and a team of scientists presented a new approach to achieve 3D motility of colloidal collectives without dispersion. The colloidal collective consisted of ferrofluidic iron colloidal particles with a diameter below 1 μm, driven by a tailored rotating magnetic field to self-assemble into a dynamic stable collective.
The team focused on optical convective flow using fluid currents for 3D drifting—bioinspired by plankton. Sun and the team discussed the methods for transitions of colloidal collectives to examine their locomotion capabilities, on water surfaces. The outcomes culminated in colloidal collectives with 3D mobility to adapt to complex environments with physical intelligence for locomotion, self-assembly and regulation.
Bimodal activation strategy
Sun and the research team adopted a bimodal actuation strategy of magnetic and optical fields to realize 3D locomotion of colloidal collectives.
In the first step, they triggered the formation of colloidal collectives by incorporating a magnetic field containing three adjustable parameters, including pitch angle, frequency, and strength. At first, in the absence of a magnetic field, the ferrofluidic colloids exhibited Brownian motion after settling.
Once energized by the tailored rotating magnetic field, they self-assembled to form small primitive collectives known as nonequilibrium colloidal collectives that continued to increase in size and merge with neighboring particles to contribute to their growth; the scientists confirmed this by using simulations.
The morphology of the colloidal collective depended on the strength and frequency of the applied magnetic field, which allowed the collective to maintain its integrity, triggering the formation and maintenance of its dynamic stability.
![Controllable transition of the colloidal collective through the air-water interface. (A) Transition of the colloidal collective from underwater to the water surface. The "M" and "O" labels indicate magnetic (f: 50 Hz, Bm: 9 mT, θ: 0°) and optical fields (λ: 808 nm, P: 5 W). (B) Colloidal collective sinks into water with an inclined posture (f: 50 Hz, Bm: 9 mT, θ: from 0° to 20°). [(A) and (B)] Scale bars, 3 mm. Credit: Science Advances, doi: 10.1126/sciadv.adj4201 Bioinspired self-assembled colloidal collectives of active matter systems](https://scx1.b-cdn.net/csz/news/800a/2023/bioinspired-self-assem-2.jpg)
Temperature gradient
The dispersed ferrofluid colloidal particles absorbed near-infrared light to convert it to heat energy, giving rise to a local temperature gradient. The temperature gradient induced a convective flow to carry the particles upward to gather into a collective with an enhanced photothermal effect. This resulted in the maintenance of a dynamically stable entity, without disintegrating.
In the absence of a near-infrared optical field, the colloidal collective cooled down with a weakened hydrodynamic force to sink progressively under gravity.
These samples therefore adjusted the optical field for convection and achieved vertical upward, hovering, and directional horizontal motion. Since the hydrodynamic force was greater than gravity, the convection pushed the collective upward vertically, allowing the colloidal collective to hover underwater. By regulating the optical field, Sun and team directed the motion of the colloid collective and adjusted their positions underwater.
Transitions through the air-water interface
The scientists investigated the ability of the colloidal collective to break through the water surface using induced convection flow; to indicate how the samples successfully exited the water by overcoming the surface tension of the water.
The colloidal collectives overcame surface tension and gravity for well-regulated transitions through the water surface to dive into water at a desired location and time. The researchers analyzed the constructs by using buoyancy, hydrodynamic force from convection, surface tension, and gravity.
![Adaptive locomotion of the microrobot collective. (A) Illustration of the microrobot collective locomotion underwater and at the air-water interface among 3D obstacles. The microrobot collectives can move underwater, maneuver on the water surface, dive into water, and make transitions between the water surface and the underwater environment. (B) Microrobot collective moves on the water surface under the magnetic field (f: 50 Hz, Bm: 9 mT, θ: 10°). (C) Microrobot collective climbs up the water meniscus under the optical field. (D) A collective crosses an obstacle with a height of 10 mm. (E) Microrobot collective passes through a channel with a diameter of 2.5 mm (f: 50 Hz, Bm: 9 mT, θ: 10°). (F) Microrobot collective crosses a gap with a width of 10 mm and climbs the high obstacle along the water-air interface. [(A) to (F)] Scale bars, 3 mm. Credit: Science Advances, doi: 10.1126/sciadv.adj4201 Bioinspired self-assembled colloidal collectives of active matter systems](https://scx1.b-cdn.net/csz/news/800a/2023/bioinspired-self-assem-3.jpg)
Sun and team explored these effects on conventional microrobot collectives to introduce spatially symmetrical interactions for locomotion underwater, and on the water’s surface. The team used magnetic and optical fields to drive the movement of such microrobot collectives on the water surface, where they climbed the water meniscus for transport driven by an optical field. Such instruments known as surface walkers can cross obstacles larger than their own size and bypass high barriers for applications in environmental science, medicine, and engineering.
Outlook
In this way, Mengmeng Sun and colleagues were bioinspired by the migration mechanisms of plankton to propel colloidal collectives to move in 3D space without boundaries. The team combined magnetic and optical fields for well-formed and regulated 3D locomotion of active colloidal collectives in an aquatic environment, with the combined optical and magnetic fields to facilitate 3D locomotion.
These sediments and colloidal systems provide a powerful process to explore the physics of self-assembly and develop a practical method to synthesize functional materials.
The living systems can form self-assembled colloidal collectives under external magnetic fields, to create structures that can be guided through spaces and interfaces, to attain unusual geometries and patterns.
Sun and team intend to investigate these collectives and their complexity for materials synthesis and design. These dual-responsive constructs can function as microrobot collectives for environmental adaptability with practical applications in biofluids with high viscosity and high ionic concentrations with broad applications in biomedical engineering.